Research Projects
:: Formation and Stability of Zwitterionic Amino-Acid Enolates
There have been many qualitative studies of the carbon deprotonation of
L-amino acid residues in peptides and proteins. For example, racemization
of amino acid residues and exchange of the α-amino proton for labeled
hydrogen from solvent have both been observed during the hydrolysis of
proteins in acidic and basic solution. Amino acid residues at proteins have
been shown to racemize faster than amino acid monomers; and, the relative
rates of racemization of different amino acid residues at proteins have
been reported. Finally, the levels of accumulation of D-aspartic acid in
the brain, tooth enamel, bones and lens protein have been determined and
shown to provide a rough measure of the age of these tissues.
By comparison, when we began our work in this area there were no
quantitative determinations of the carbon acid pKa of the α-amino
protons of amino acid residues at peptides or proteins. These carbon
acidities are required for any full characterization of the chemical
reactivity of these important compounds. They are relevant to the mechanism
of racemization of amino acid residues in tissue, fossils, marine sediments
and agricultural produce. They are also of significant chemical interest,
because of the insight that they would provide into the effect of the
different functional groups present in peptides and proteins on the acidity
of α-carbonyl protons.
Our work in this area has provided a thorough characterization of the
acidity of the α- amino protons of glycine and derivatives of glycine
including di-and tripeptides of glycine. We have also determined the
acidity of α-amino protons of proline methyl ester. These data are
important in their own right; and they have provided important insight into
the mechanism for catalysis of proton transfer reactions of α-amino
acids. See
(a)
Journal of the American Chemical Society, 122, 9373-9385
(2000) and (b)
Journal of the American Chemical Society, 124, 8251-8259,
(2002).
(1) Scheme 1 shows a comparison of nonenzymatic enzyme-catalyzed
deprotonation of proline, using our data for the thermodynamic barrier to
enolization. The principal barrier which must be lowered to achieve the ca.
19 kcal/mol stabilization of this transition state effected by enzymes such
as proline racemase is the 27 kcal/mol thermodynamic barrier to conversion
of the amino acid zwitterion to the zwitterionic enol (Kenol = 10-20,
Scheme 1). This large barrier to keto-enol tautomerization of proline
zwitterion in water can be reduced at an enzyme active site provided the
enzyme binds the enol zwitterion intermediate more tightly than it binds
the substrate amino acid zwitterion, so that (Kd)S >>(Kd)I (Scheme 1). Our
work has suggested an important mechanisms by which such selectivity for
binding of the intermediate of the reaction catalyzed by proline racemase
may be achieved.
(2) We have to reported extraordinarily efficient catalysis of
deprotonation of the α-amino carbon of glycine
methyl ester by the simple ketone acetone and shown that this catalysis is
the result of a 107-fold larger acidity constant KCH
for carbon deprotonation of the iminium ion adduct IH+
(pKCH = 14) than for deprotonation of N-protonated glycine
methyl ester GH+ (pKCH = 21). See Journal of the American Chemical
Society, 123, 7949-7950 (2001).
(3) The chemistry of pyridoxal 5'-phosphate (PLP) is thought to be well
understood, and we expected that the PLP analog 1 would be an effective
catalyst of the deuterium exchange reaction of glycine. We were therefore
surprised when 1H-NMR analysis of the reaction of 10 mM 1 with 100 mM
glycine in D2O at pD 7.0 and 25 C showed the loss 1 in a clean first-order
reaction (kobsd = 4.3 x 10-5 /s) to form an equilibrium mixture that
contains 3% of 1 and two isomeric products in a ratio of 2.1:1.0 that were
identified as adducts of glycine to 1. There is no detectable (<1%)
transfer of deuterium from D2O to glycine or formation of
2'-deoxypyridoxamine from a transamination reaction during the first four
halftimes of the reaction of 1. We find instead that the reaction between 1
and glycine gives a quantitative yield of 2 and 3 from a Claison
condensation reaction (Scheme 2). The Claisen condensation reaction between
1 and glycine escaped characterization in earlier work, because of its
strangeness in comparison to textbook PLP-catalyzed reactions. Water is a
poor solvent for Claisen condensation reactions, because this solvent is
moderately acidic and will rapidly protonate basic enolates of simple
carboxylic acid derivatives. The formation of 2 and 3 in good yields from
addition of the glycine enolate 4 to 0.1 mM 1 in water is a consequence of
the unprecedented large selectivity of 4 for addition to 1 in a protic
solvent that contains 50 mM of a buffer acid of pKa 7. [In Preparation]
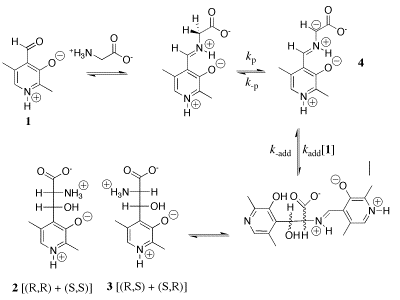
>> Back to top
|

|