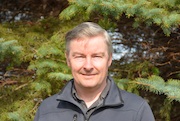
D. Scott Mackay
Professor
Department of Geography
State University of New York
105 Wilkeson Quadrangle
Buffalo, NY 14261 USA
Phone: +1-716-645-0477
Fax: +1-716-645-2329
dsmackay at buffalo dot edu
Home
|
Ecohydrology
Group's Projects
Current Projects
Expansion and stimulation of the
rhizosphere during hydraulic
redistribution. Department of Energy,
Environmental Systems Science.
With Richard Marinos (University of
Buffalo), Angela Possinger (City University
of New York), and unfunded collaborators Jim
Moran and Adam Mangel (PNNL), September 2022
to July 2025.
Plant root systems can act as conduits for
water from wet regions of soil to dry
regions of soil, a phenomenon known as
hydraulic redistribution (HR). HR plays a
variety of ecological roles, including
preventing root desiccation, increasing
plant resilience to drought, and providing
water to soil organisms. This is one of the
many ways that roots shape the soil
environment. Another important way is
through the release of sugars and other
photosynthesis products into the soil, which
fuels the metabolisms of soil microbes. This
process is called exudation and is known to
be an important control on soil microbial
activity and soil health.
In this project, we propose to examine the
interplay between HR and root exudation. We
hypothesize that HR will increase exudation
during drought conditions, acting to
stimulate microbial activity and sustain
soil biogeochemical processes during
drought. To examine this hypothesis, we will
perform an integrated suite of experiments
and modeling to determine how HR modifies
the quantity and quality of root exudates
and how this in turn impacts microbial
activity in the soil. We will quantify
changes in exudate quantity and composition
using state-of-the-art high-resolution mass
spectrometry techniques. We will determine
if HR alters spatial patterns of root
exudation using mass spectrometry imaging.
Further, we will track the transport and
fate of
exudates in the soil with isotopic imaging
techniques. These experiments will inform
two cutting-edge models. The first model
simulates the transport and microbial uptake
of exudates from the root into the soil at
the scale of individual roots. The second
model simulates the incorporation of these
root-scale processes into a model of whole
plant-soil ecophysiology, allowing us to
determine how HR impacts plant performance
and soil health. Results from this study
will enhance our ability to predict
ecosystem responses to drought and better
plan for extreme climatic events.
RESEARCH: Predicting Genotypic Variation
in Growth and Yield under Abiotic Stress
through Biophysical Process Modeling.
National Science Foundation, Plant Genome
Research Project. With Brent
Ewers (PI, Wyoming), Daniel Kliebenstein (UC
Davis), C. Robertson McClung (Dartmouth),
and Cynthia Weinig (Wyoming), September 1
2016 to February 28 2025.
Rising demand for high quality food crops
due to increasing world populations along
with more likely temperature and drought
stress requires further crop improvements
from breeding programs. A major limitation
to these programs is an understanding of how
the genetic information affects the
characteristics of plants that improve the
amount of edible portions. Moreover, the
predictive understanding is even less if the
plants are placed in new, stressful
environments like low rainfall or high
temperature. A likely avenue of inquiry to
improve breeding is to better connect how
information stored in genes becomes traits
of plants that combine their biology, such
as photosynthetic rate or the amount of
resources allocated to an edible root, with
the physical world, such as the amount of
water available or an excessive heat wave.
These connections are currently often made
in a way that requires new data collection
for every new crop plant or environment such
as a new soil, new temperature range or even
a new improved line that shows variation in
the amount of resources the plant allocates
to an edible root. This continuous need for
new information ultimately slows down the
breeding program and the ability of plant
scientists to quickly respond to the needs
of society. This project will test a new
approach that uses large amounts of data to
calculate the probability that a particular
plant characteristic will be displayed by a
given plant line under various environmental
conditions. Specifically, the project will
measure plant performance continuously by
sending electrical pulses through plants,
integrating the data generated with large
data sets that show which genes are active
as well as the level of biologically
relevant molecules that contribute to major
metabolic pathways within the plants at any
given time. This new approach requires high
performance computing to test many times how
the probability of phenotypic improvement in
the crop may occur. These high performance
computing approaches will become a core part
of a modern, competitive workforce. In this
regard, the project will provide workshops
for high school teachers in the use of high
performance yet open source computing tools
in their classrooms. In addition, the
project will develop experimental and
computational modules in biological and
quantitative learning for students in grades
6-12 using the highly successful Wisconsin
FastPlants system
(http://www.fastplants.org/).
With increasing world populations, genetic
advances to improve crop growth, yield and
resistance to abiotic stress are a pressing
need. Limiting the speed of crop improvement
is a crucial knowledge gap regarding
biophysical processes that modulate the
relationship between the genome and phenome,
hindering the ability to predict the
phenotype of novel genotypes in novel
environments. As a first step towards
bridging this gap, a combination of
high-throughput phenotyping and biophysical
process modeling will incorporate allelic
variation at key genes affecting plant
carbon metabolism, hydraulics, and resource
allocation, all of which are known to impact
drought- and heat-stress resistance in
plants. Variable selective pressures during
crop diversification have caused extensive
phenotypic variation among B. rapa crops,
making it an excellent study system to both
connect organ-level measures both down to
the level of transcriptomic and metabolomic
phenotypes and up to yield and to test
predictive process models. Process models
will be developed and refined using the
mechanistic links that connect cell
processes and ultimately whole plant
physiology to regulatory intermediates such
as metabolites and gene transcripts. If
successful, the models developed will enable
prediction of whole-plant stress-response
phenotypes in heterogeneous genotypes and
environments. The goals of the project are
to: 1) deploy a novel high-throughput and
real-time phenotyping method to measure diel
physiological dynamics in eight B. rapa
parental Nested Association Mapping (NAM)
lines under drought- and heat-stress
conditions; 2) predict yield in a
Recombinant Inbred Line (RIL) population of
B. rapa using a biophysical process model of
carbon metabolism, hydraulics and resource
allocation to test systems-level links
between circadian, transcriptomic,
metabolomic, and physiological QTL; and 3)
test the predictive ability of the
biophysical process model under heat- and
drought-stress environments using the RIL
population used in Aim 2. All data and
resources generated in this project will be
made accessible to the public through
long-term open access repositories such as
Project Github and the NCBI Short Read
Archive.
A systems analysis of plant growth
promotion by the rhizosphere microbiome.
National Science Foundation, Plant Genome
Research Program. With Cynthia Weinig (PI,
Wyoming), Marc Brock and Brent Ewers
(Wyoming), Lois Maignien (MBL), Mike Covington
and Ian Korf (UC Davis). Project period: June
1, 2015 to May 31, 2023.
Soil microorganisms serve a range of ecosystem
services that promote plant growth under
stressful abiotic conditions, including
nitrogen limitation. Yet, soil microbial
communities represent a largely untapped
source for yield improvement in crop species.
Analyzing plant gene expression (when
different genes are turned on and off) and
physiological responses to diverse soil
micro-organisms will provide insights as to
the mechanisms by which microbes enhance plant
growth. Further, modeling of plant gene
expression, hydraulic, and gas-exchange
responses to the soil microbial environment
can provide a predictive understanding of
plant growth promotion by microbes under
variable nitrogen (fertilizer) amendments.
When grown with an intact vs.
reduced set of soil microbes, crops of Brassica
rapa upregulate gas-exchange and
increase biomass accumulation up to two-fold,
suggesting one physiological link between
plant gene expression responses to soil
microbes and eventual plant biomass
accumulation. Because B. rapa
is domesticated as root, leaf, and oilseed
crops, mechanisms of growth promotion
characterized in this species could translate
to a range of other crops. Using sequencing of
plant and soil microbe DNA and analyses of
plant responses, the research will
characterize mechanisms of plant growth
promotion by soil microbes and test predictive
systems models. Soil microbes reflect a
biological continuum between the plant and the
environment. While much is known about the
identity and functions of plant species within
natural and agricultural ecosystems, much less
is known about biogeographic distributions,
identity, and functions of beneficial
rhizosphere microbes and their role in
alleviating soil N stress. The current
research fills these knowledge gaps. Further,
modeling will provide predictive links from
genomic, transcriptomic, and physiological
features to plant growth promotion in response
to beneficial soil microbes.
Past Projects
Collaborative
research: Integrating plant hydraulics with
climate and hydrology to understand and
predict responses to climate change.
National Science Foundation, Integrated
Ecological Physiology program. With John Sperry
(PI, Utah), Paul Brooks (Utah), and William
Anderegg (Princeton). Project period: May 15,
2015 to April 30, 2019.
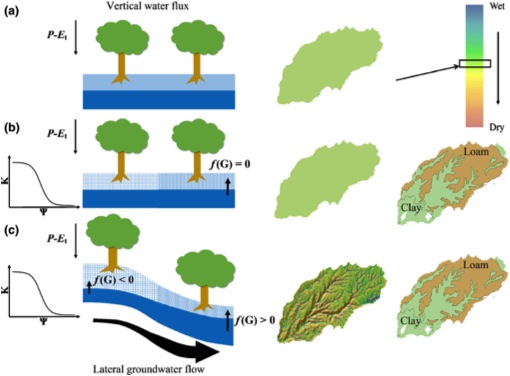
Droughts threaten the health of the nation's
forest resources by reducing tree growth and
increasing mortality from multiple causes
including fires and insect attack. This project
will determine when and where forests are most
at risk from drought-induced mortality in the
western USA under current and future climate
scenarios. Plant drought responses will be
predicted from a theory of water supply and
demand that is based on the physics of water
flow. When plants are photosynthesizing they are
also losing water by evaporation through the
stomatal pores that take up CO2 in their leaves.
The lost water is replenished by a passive
wicking process that pulls water from the soil
into roots and up dead, water-filled xylem
tubes. Drought-induced failure of this water
transport limits the possible rate of water
supply, which by theory is associated with
reduced water demand caused by closure of
stomatal pores. Consequently, prolonged droughts
reduce growth and transport capacity, creating
persistent metabolic stresses that increase
mortality by the "chronic stress
hypothesis". A computer model will combine
the supply-demand theory with climatic and
landscape causes of drought stress, including
precipitation, groundwater hydrology, and
atmospheric humidity. The model and chronic
stress hypothesis will be tested in greenhouse,
plantation, and natural stands, focusing on
trees of the intermountain west. Predictions for
the region's forests will be made from climate
projections. Forecasts will inform decisions on
land management. Educational versions of the
model will illustrate the combined roles of
climate and groundwater hydrology on plant
health. The project is a regionally focused
trial of an approach that can be applied
nationally and globally.
Terrestrial
Regional Ecosystem Exchange Simulator
(TREES)
We have developed an object-oriented
ecosystem process model for application at
leaf, organism, stand, and larger scales. It
is being used to understand coupled hydraulic
and carbon uptake/utilization drivers of tree
mortality, forest responses to disturbance
such as bark beetles, effects of drought on
water, carbon, and biogeochemical processes,
and key processes for scaling to flux towers,
watersheds, and regional scales. It is also
being used along with Bayesian analysis to
understand model/data complexity requirements,
uncertainty analysis, and error propagation.
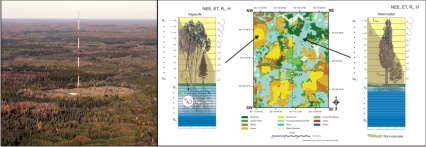
Improving prediction of climate
change impacts on wetland-rich
landscapes: Testing model mechanisms
with flux-data assimilation at multiple
sites. Department of
Energy, NICCR program.
Prediction of climate change impacts
on terrestrial carbon fluxes is highly
uncertain. Upland ecosystem models, even when
constrained with flux tower data, fail to
explain interannual variability in CO2 fluxes
in the upper Midwest. One possible reason is
lack of model mechanisms for wetland
biogeochemistry and hydrology, where fluxes
would be expected to vary with changes in
depth to saturation. Wetlands are expected to
be highly sensitive to climate change. We
propose to develop a wetland-landscape model
and assimilate long-term multiple flux tower
observations to simulate wetland and upland
mechanisms simultaneously, with evaluation
against unassimilated flux observations. Model
evaluation is typically limited to single
sites and extrapolation of these results
across larger regions is questionable. This
research will improve understanding of
carbon-rich forest-wetland landscape response
to climatic change.
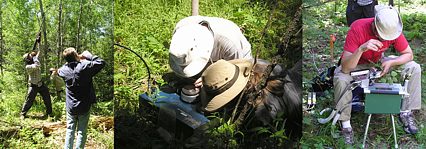
Collaborative
research: Restricted plasticity of
canopy stomatal conductance: Conceptual
basis for simplified models of canopy
transpiration. National Science Foundation,
Hydrologic Sciences Program.
Project
Web site
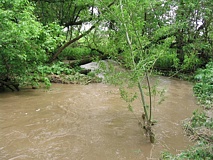 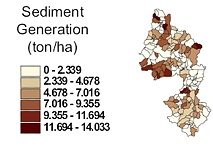
Measuring and modeling the
source, transport and bioavailability of
phosphorus in agricultural watersheds.
Environmental Protection Agency, STAR program.
Project web site
We will measure and model the
sources, transport, and fate of bioavailable
phosphorus (BAP) in the mostly agricultural
604-km2 watershed of Lake Mendota near
Madison, Wisconsin. This well-known eutrophic
lake is impacted by phosphorus (P) loading
from agricultural sources, including row
crops, dairy operations, and land applications
of manure and commercial fertilizers. The
research will compliment an on-going
nonpollution abatement program targeted at
reducing P inputs to the lake through improved
management of these agricultural sources. Our
multidisciplinary team provides the expertise
in social science, outreach, environmental
modeling, environmental chemistry, hydrology
and sediment transport, environmental
engineering, and limnology – all of which are
needed to accomplish our “systems” goals. Our
focus is on the scale-dependent processes that
link agricultural P sources to watershed
export of BAP. Our plan involves six major
objectives: 1) Quantification of the effects
of manure management on runoff BAP; 2)
Measurement of the amounts, spatial patterns,
and transport of sediment and BAP in channels
and streams acting as routes for transport
through the watershed; 3) Quantification of
the in-stream processes governing the fate and
transport of sediment P; 4) Evaluation and
improvement of modeling tools (APEX, SWAT) for
assessing P transport over a wide range of
spatial scales; 5) Determination of the
relation of BAP losses to the scale of animal
operations; and 6) Integrated outreach with
stakeholders, agency partners and other
researchers through farmer-feedback
procedures, agency assessments and model
refinement. Knowledge gained will be crucial
for effective state and national TMDL
development and implementation.
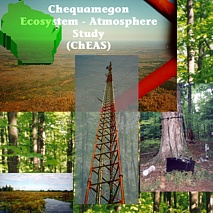 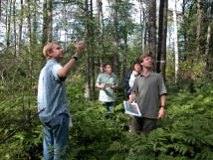
Chequamegon
Ecosystem-Atmosphere Study (ChEAS)
ChEAS is a NSF-funded
interdisciplinary research collaboration
network project aimed at understanding
land-atmosphere exchange of cabon, water, and
energy at one of the tallest AmeriFlux towers,
located near Park Falls, Wisconsin. The lead
institution for the project is Penn. State
University; Dr. Mackay is one of 6 members of
the ChEAS steering committee.
Link
to ChEAS
Adaptive Parameter Restriction and Selection
(APReS)
We are developing a general framework
for the analysis of uncertainty in simulation
models. Our approach is motivated by methods
such as GLUE and Parato Optimality. The
premise of APReS is that parameter uncertainty
and even model structure uncertainty are
interpretable in terms of fuzzy sets. A
nonmonotonic reasoning approach is supported
to allow for the evaluation of simulations
based on multiple criteria in different
combinations and priorities. APReS has been
applied to simulation of streamflow in
forested watersheds, transpiration at
hillslope to watershed scales, and to
transpiration at individual tree and
whole-stand scales. It is currently being used
as one of numerous analytical tools for
projects funded by NSF and EPA.
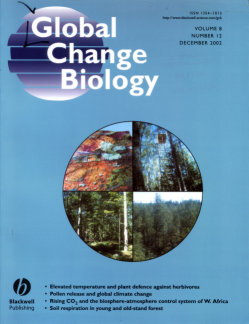 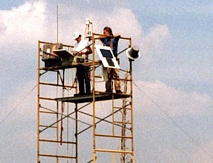
Long-term water flux changes
from converting old-growth pine forests to
hardwood forests in northern Wisconsin.
NASA, Land Surface Hydrology Program,
NAG5-8554, 1999-2003, Mackay (PI).
Highlights and milestones of the Land
Surface Hydrology Project were:
1. A comprehensive database of
vegetation, water fluxes, micrometeorology,
remote sensing, soil moisture, tower flux, and
process-based models were used in the first
successful scaling-up exercise at the WLEF
tower (Mackay et al., 2002; Ahl et al.,
2004b).
2. An essential bridge was developed between
leaf and tower flux measurements with
measurements of leaf water potential and
transpiration among seven key species in the
WLEF tower footprint (Ewers et al., 2002;
Ewers et al., 2004).
3. Accurate simulations of transpiration
models and parameterization for the
heterogeneous forests around WLEF (Mackay et
al., 2003a,b; Samanta and Mackay, 2003).
4. We developed a parameterization scheme to
use MODIS land surface temperature to
estimates canopy stomatal conductance for
simulation transpiration and photosynthesis
(Mackay et al., 2003c; Mackay et al., 2004).
Other
Past Projects
-
Distributed
parameter non-point source pollution
modeling in nested watersheds: Guide to
implementing legislated surface water
quality restrictions in Wisconsin.
Hatch/McIntire-Stennis, October 2000 to
September 2004, Mackay (PI).
-
Context
elicitation to support the semantic
integration of environmental models.
University of Wisconsin Graduate School
Fall Competition, July 2000-June 2001,
Mackay (PI).
-
Effects of
parameter spatial aggregation on
agricultural non-point source pollutions
models. US EPA (Pass-through from
Wisconsin Department of Natural
Resources), 1999-2000, Mackay (PI).
-
Remote
sensing teaching and research in support
of creating a vision for the environment
as a whole. NASA, Centers of Excellence in
Remote Sensing, NAG5-6535, 1997-1999,
Mackay (PI).
-
Coupling
forest ecosystem process-based models to
groundwater models: tools to guide natural
resource management in northern Wisconsin.
Funded by McIntire-Stennis, 1997-2001,
Mackay (co-PI) with Gower (PI).
-
Affiliated
Research Center (ARC). NASA., Mackay (one
of several co-investigators) with
Lillesand (PI), 1996-2001. ARCII. NASA,
Mackay (one of several co-investigators)
with Lillesand (PI), 2001-2006.
-
Scaling
spatial simulation of forest disturbance
on watershed processes, University of
Wisconsin Graduate School Fall
Competition, 1997-1998, Mackay (PI).
-
Characterization
of evapotranspiration and forest water
relations in northern Wisconsin.
University of Wisconsin Graduate School
Fall Competition, July 2001-June 2002.
-
Chequamegon
Ecosystem-Atmosphere Study. National
Science Foundation, Division of Biological
Sciences, Research Collaboration Network
Program. Mackay one of several
co-investigators with Ken Davis, Penn.
State (PI), January 1, 2002 to December
31, 2006.
-
Development
and Evaluation of TMDL Planning and
Assessment Tools and Processes. USDA
CSREES Regional Project DC 00-02, Theo
Dillaha, U. Virginia (PI). Mackay, one of
two UW-Madison representative, October 1,
2001 to September 30, 2006.
-
Quantifying
non-point source phosphorus losses from
field to watershed scales. University of
Wisconsin Graduate School Fall
Competition, Interdisciplinary, July
2002-June 2003; co-investigator with K.G.
Karthikeyan as PI.
-
WebGrant
Award. University of Wisconsin Division of
Technology instruction web page
improvement award.
|